Research Areas
Research Areas
The various projects proposed for SFB 953 Synthetic Carbon Allotropes are structured in three research areas and two central projects. Research Area A (Synthesis and Functionalization) provides the materials basis of the SFB. Both chemical functionalization of known synthetic carbon allotropes and development of new carbon modifications lie at the forefront. The next level within the process chain is the systematic investigation of physical and materials properties and the development of concepts for device fabrication. This is guaranteed by the close interaction with Research Area B (Electronic, Optical and Structural Properties), where systems synthesized in Research Area A will be studied in great detail. This highly integrated and interdisciplinary approach of the SFB also necessitates a close connection with Research Area C (Theory). Both classical and quantum mechanical calculations provide the basis for an in-depth understanding of reaction mechanisms, stability, electronic and optical properties, structural and mechanical properties of synthetic carbon allotropes and their derivatives. Moreover, theory will provide some of the most valuable design principles for the exploration of hitherto unknown forms of carbon. Finally, developing and strengthening fundamental and applied carbon-allotrope research requires strong support from highly sophisticated analysis and structural characterization, which is provided by the two Z Projects on tandem mass spectrometry and high-resolution electron microscopy. Making use of the latest developments in analysis of carbon materials and advanced instrumentation, like dedicated mass spectrometry and aberration-corrected TEM, the goal of the two Z Projects is to contribute to an atomic scale understanding of structure and structure-property relationships of carbon allotropes and related devices.
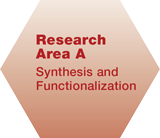
The seven PIs involved in synthesis represent a fine balance between targeting fundamental goals and perspectives for applications. From a fundamental perspective, new methodologies are proposed that will significantly expand the range of known allotrope structures and derivatives. At the same time, mechanistic and structure-property studies also feature prominently in these projects with the aim of creating a firm foundation for the discovery of new molecular structures. In several cases, novel methods are developed to adequately characterize the newly formed allotropes using, for example, Raman spectroscopy/microscopy or ultracentrifugation. It is particularly noteworthy that many of the molecules, networks, and allotropes proposed in the projects of Research Area A represent ambitious synthetic targets that will greatly expand the boundaries of carbon-allotrope chemistry.
Achieving these synthetic challenges will be aided greatly by collaborations with theorists in Research Area C and analysis in the two scientific Z Projects, who offer guidance on the expected stability of new targets, mechanistic interpretation, as well as structural considerations for the novel systems.Once synthesis, purification, and characterization are complete, each of the eight synthetic projects proposes well-defined target systems to serve as components in devices or as substrates for analysis with collaborators in Research Area B. Carbon allotropes offer nearly endless opportunities as new materials and functionalization can be selectively engineered to offer structures for applications in all-carbon architectures for solar cells, molecular wires, field-effect transistors, switches, as well as other optoelectronic devices or catalytic and sensoric applications. The molecules from Research Area A projects will be integrated into electronic, optical, and structural applications in Research Area B using strong ties between experimentalists and theorists in Research Area C, many of which have been developed and cultured in the first funding period and are expanded to incorporate the new members joining in the next funding cycle.
In the broadest sense, objectives from Research Area A are grouped into four categories, 1) Fullerenes, 2) Carbon Nanotubes, 3) Graphene, and 4) Novel Carbon Allotropes (including heteroatom-doped graphene-like structures, carbon nanodots, polyynes/cumulenes/carbyne, and graphynes/graphdiynes). The distribution of projects is shown in the Table, and specific topics are summarized as follows
Project A1 Unifying Concepts for the Chemistry of Synthetic Carbon Allotropes by PI Hirsch will develop an integrated approach for the chemistry of synthetic carbon allotropes (SCAs): fullerenes, carbon nanotubes, and graphene. Both covalent (SCA halogenides, carboxylates, sulfonates, phosphonates and Lewis-acid adducts) and non-covalent (dispersion, exfoliation, sorting of SCAs with amphiphilic rylenes) chemistry will be refined and optimized. The nature of the SCA building block, its topology and arrangement (e.g., surface supported monolayer, bilayer etc.) on reactivity will be explored, with an underlying focus on reaction and/or supramolecular mechanisms. Synthetic studies will evolve concurrently with newly developed analytical tools such as scanning Raman spectroscopy (SRS), scanning Raman microscopy (SRM) and, TGA/MS/GS, along with partners contributing, for example, quantum mechanical calculations and transport measurements. Finally, a new initiative will be developed within the collaborative research center, namely the investigation of All-Carbon Architectures as a new field in materials science.
Project A2 Graphyrins – Graphene-Porphyrin Hybrids by PI Jux will use the porphyrin skeleton as a platform to synthesize graphene-like carbon networks selectively doped with nitrogen atoms (called graphyrins). Smaller graphyrins would posses s bowl-shaped structures and offer a class of substrates that should form complexes within the concave surface, including fullerene complexation, metal incorporation, and axial coordination. Larger graphyrins will be assembled by clever attachment of polycyclic aromatic building blocks to a porphyrin core, and the products will mimic, for example, the shape and geometry of carbon nanotubes. Hexaphenylbenzenes (HPBs) and hexa-peri-hexabenzocoronenes (HBCs) will be assembled to serve as molecular wires and carbon nanoribbons. Graphyrins, HPBs, and HBCs will be functionalized and studied with a focus on electron-transfer properties as well as interactions with various surfaces.
Project A3 Process Design Strategies for Advanced Carbon Allotropes and Hybrid Nanostructures by PI Peukert outlines the scalable and size-selected synthesis for carbon nanodots (CNDs) by solvothermal methods. Single-walled carbon nanotubes (SWCNTs), on the other hand, will be synthesized in a fluidized bed reactor utilizing tailored non-ferromagnetic catalysts to allow separation of metallic and semiconducting SWCNTs. Hybrids formed from carbon allotropes and nanoparticles by wet-chemical methods and surface-assisted growth from the gas phase will also be established. The development of new techniques for characterizing the new SCA materials will play a central role, including electrospray-differential mobility analysis-mass spectrometry and multi-wavelength analytical ultracentrifugation. Combining carbon allotropes with nanoparticles will be used to tune properties of the new SCAs for applications in solar cells, fuel cells, batteries, and catalysis.
Project A4 The Next Generation of Carbon Allotropes: On the Way to Carbyne and Graphyne by PI Tykwinski focuses on the synthesis of the new carbon allotropes carbyne and graphyne, which are derived from sp-hybridized carbon building blocks (i.e., acetylenes and cumulenes). Monodisperse polyynes and cumulenes will serve as models for the allotrope carbyne, and these molecules also form the basis of molecular wires for single molecule conductivity measurements. Understanding the similarities and/or differences between the polyyne and cumulene versions of carbyne is a primary focus. Acetylene-rich small molecules will be exploited as precursors for the both surface and solution-state synthesis of new carbon allotropes such as graphyne and graphdiyne, which will be studied as unprecedented organic (semi)conductors.
Project A5 Molecular Fragments of Heteroatom-Doped Carbon Allotropes by Chemical Synthesis by PI Kivala will construct defined polycyclic aromatic hydrocarbon networks that are selectively doped with nitrogen and phosphorus. Efficient electronic communication between the heteroatom and the surrounding sp2-carbon network will be used to modify optoelectronic materials properties in comparison to the all-carbon counterparts, which renders the targeted compounds quite promising as functional materials. On the fundamental side, the parallels and differences in properties of analogous nitrogen- and phosphorus-doped sp2-carbon scaffolds will be investigated. Alternative synthetic methods will be explored, such as flash vacuum pyrolysis (FVP), as well as surface-assisted reactions followed by scanning probe microscopy, toward the fabrication of highly ordered carbon networks from carefully designed molecular precursors.
Project A6 Atomically Precise Synthesis of Carbon Nanotubes and Related Structures by PI Amsharov will use surface assisted cyclodehydrogenation (SACDH) of specially designed precursor molecules for the preparative fabrication of helically pure and defect free single-walled carbon nanotubes (SWCNTs). Surface synthesis offers many options toward forming isomerically pure SWCNTs, with virtually any desired chirality, based on modification of the cap structure (including size, chirality, and periphery geometry) and the surface used (different metals, different morphology). The synthesis of metallic armchair and zig-zag nanotubes of various diameters will also be addressed. In addition to all-carbon nanotubes, the synthesis of functional SWCNTs based on porphyrin building blocks will be developed, to form heteroatom structures such as fullerene-like system and carbon nanocones. Finally, the SACDH technique for bulk CNT production will be optimized to use chemical vapor deposition.
Project A7 Mechanically Interlocked Synthetic Carbon Allotropes by PI von Delius will explore the synthesis of mechanically interlocked materials (MIAs) based on carbon nanotubes and fullerenes. In spite of their unique dynamic properties and application prospects, little is known about MIAs based on SCAs. The reversible formation of disulfide bonds will guide the assembly of macrocycles around single-walled carbon nanotubes to give polyrotaxanes that can deliver solution-processable products. Toward the realization of inter-SCA architectures, an unprecedented series of rotaxanes will be formed that feature a fullerene to function as a supramolecular binding site along the axle, as well as bulky stoppers at the ends. A [10]cycloparaphenylene ([10]CPP), representing a small synthetic segment of a SWCNT will be used as the macrocycle. The [10]CPP ring will be free to shuttle along the axle, providing unusual mechanochemical and optoelectronic properties.
In conclusion, the methods, molecules, and materials proposed in Research Area A form the fundamental basis for the discovery of new devices and applications based on carbon allotropes. The synthesis and study of these molecules, however, can only be accomplished efficiently under guidance from theorists in Research Area C projects, i.e., these cooperations will continually guide the selection and structure of the synthetic targets. On the other hand, the goals and discoveries described in Research Area A also rely on the synergistic relationship with the characterization studies of Research Area B and the Z Projects. In nearly all cases, state of the art analysis of electronic, optical and structural properties will be an integral aspect of molecular design in Research Area A.
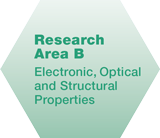
Carbon allotropes are a fascinating material class with outstanding potential for both ground-breaking science and novel optoelectronic applications. Specifically, the various dimensionalities of carbon-allotrope molecules open new interdisciplinary research challenges for physicists, chemists, and materials scientists. Semiconducting or metallic carbon allotropes are available as 0D point structures (fullerenes), 1D rods (nanotubes), 2D sheets (graphenes), while 3D bulk structures are generated via thin films from fullerenes, nanotubes, etc., or blends thereof.
Novel materials synthesized in Research Area A will be studied and characterized in great detail with respect to their fundamental properties in Research Area B. Experimental investigations will be accompanied by theoretical calculations and simulations (Research Area C) and further supported by advanced analysis (Research Area Z). Based on these findings, electronic and opto-electronic devices will be designed, processed, and investigated. As a result of these cooperative efforts and activities, selected projects of Research Area B will aim at novel devices based on fully characterized novel carbon allotropes.
The individual eight projects of Research Area B will work predominantly on the investigation and characterization of fullerenes, nanotubes, graphenes, and innovative carbon allotropes with novel properties. A special focus across all projects will be placed on exploring organic, doped, and undoped SCAs. The Table gives an overview of the material systems, which will be mainly investigated in the respective research groups. The fundamental electronic, optical, and structural investigations of SCAs will play a major role within this part of the collaborative research center. The fields of investigation by the individual partners are summarized in the Table.
Project B1 Opto-Electronic Properties of Semiconducting Carbon Allotrope Hetero-Junction Composites: Towards “All-Carbon Allotrope Architecture” Devices by PI Brabec aims to design, characterize, and investigate the opto-electronic properties of all-carbon allotrope hetero-junctions (HJs) consisting of solubilized graphene / SWCNTs (as w ell as r-GO) mixed with n-type fullerenes and being processed from solution. All-carbon allotrope solar cells and photodetectors will be realized and the film formation, the charge carrier dynamics, and the photostability of these composites will be investigated as a function of their composition as well as their microstructure.
Project B3 Design and Assembly of Carbon-Allotrope-Based Devices by PI Halik will develop methods for a defined 3D-assembly of functional carbon allotropes as a key enabler for prospective all-carbon-based electronics. Two concepts will be pursued, where the first targets a layer-by-layer assembly of functionalized allotropes with covalent (e.g., click chemistry) or non-covalent (e.g., Coulomb forces) driving forces and the second based on a concerted 3D-assembly of particular functionalized SCAs via nano-phase separated block-copolymer matrix support.
Project B4 High Resolution Scanning Probe Microscopy/Spectroscopy of Functionalized Carbon Allotropes by PI Maier aims at producing surface supported carbon allotropes and understanding their growth, structural, electronic, and mechanical properties using high resolution scanning probe microscopy and spectroscopy at the atomic scale. In particular, graphyne, heteroatom-doped graphene-like networks, wires, and nanocones as well as the non-covalent functionalization of graphene will be in the focus.
Project B7 Tuning the Surface Chemical Properties of Graphene by PIs Steinrück/Papp aims at the chemical modification of supported graphene, that is, covalently functionalized graphene via in-plane functionalization by substituting carbon atoms in the graphene plane (heterographene) and out-of-plane functionalization through attaching functional groups above or below the graphene plane. Furthermore, the formation and reactivity of metal clusters on graphene surfaces will be studied, as well as reactions of intercalated gases between graphene and the supporting substrate. In addition, the chemistry of carbon allotrope formation on surfaces and the interaction of large molecules with carbon allotropes will be investigated.
Project B8 Graphene and Organic Molecules: Transport Experiments by PI Weber aims to carry out unprecedented experiments that combine single-molecule junctions, optical excitation, and optical single-molecule spectroscopy by using the ultraflat and transparent epitaxial graphene electrodes. Further, the electronic and optical properties of stacking faults will be extensively investigated and their manipulation is targeted.
Project B10 Photophysical Characterization of Synthetic Carbon-Allotrope-Based Materials by PI Guldi will use advanced photophysical means to probe photon and charge management as well as molecular wire behavior in All-Carbon Architectures. The work is rounded off by efforts towards supramolecular charge transfer chemistry to interrogate structure and properties of extended defects and to fine tune the electronic properties of all-carbon nanostructures with spatial resolution.
Project B11 Synthetic Carbon Allotropes as Core Material in Strong-Field and Low-Energy Electron Physics Experiments by PI Hommelhoff will investigate the suitability of synthetic carbon allotropes as functional building blocks in ultrafast and low-energy electron physics. Intense phase-controlled laser pulses will be utilized to investigate sub-cycle (attosecond) current control in graphene nanogaps as well as in graphene-based molecular junctions, while large free-standing ultraclean graphene flakes and carbon nanotubes will be investigated towards eV-level electron amplitude beam splitter operation and ultrafast light matter interaction, respectively.
Project B12 Controlling Electronic Properties of Individual Synthetic Carbon Allotropes by Physical and Chemical Routes by PI Krstić will explore the common relevance of strain on chemistry and electronic properties in SCA materials. A combination of in operandi methods is employed with emphasis on chemical modification and resulting transport properties. In a second line of research, the relevance of (local) strain as a gauge field on layered SCAs is explored.
A second, equally important, aspect of Research Area B are device-related investigations and developments. The concept of doping carbon allotropes and forming heterojunctions of various dimensionalities are at forefront. Advanced device concepts and investigations will be implemented in various thin-film electronic applications such as carbon allotrope-based solar cells, transistors, sensors, or conductors. Furthermore, the unique material strength in SCAs as well as the excellent material control allow novel experiments that will grant unprecedented experimental access to new phenomena. An overview on device-oriented activities of the single projects is summarized in the Table.
Concluding, Research Area B will continue to be well connected with Research Area A, C, and Z and is designed to give essential input to the chemistry partners (as request for specifically designed novel materials). Further, Research Area B will deliver experimental data relevant for and required by the theory partners. We will go significantly beyond the state of the art in the characterization and investigation of novel carbon allotropes and in designing and realization of novel devices. Employing SCA materials, which provide materials with unprecedented properties, novel experiments, and insights will become possible. All these reach far beyond the SCA material class.
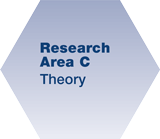
Project C1 Large Scale Simulations on Carbon Allotropes by PIs Clark/Meyer concentrates on what are traditionally considered the “modeling” aspects of the chemistry of carbon allotropes; large-scale calculations and molecular dynamics. To this end, fast approximate molecular orbital (MO) techniques will be developed for and applied to questions that arise in the experimental projects. Both classical and quantum mechanical molecular-dynamics simulations will be used to sample conformations of flexible systems and to determine reaction paths using the metadynamics technique.
Project C2 Large Scale Simulations on Carbon Allotropes by PIs Clark/Meyer concentrates on what are traditionally considered the “modeling” aspects of the chemistry of carbon allotropes; large-scale calculations and molecular dynamics. To this end, fast approximate molecular orbital (MO) techniques will be developed for and applied to questions that arise in the experimental projects. Both classical and quantum mechanical molecular-dynamics simulations will be used to sample conformations of flexible systems and to determine reaction paths using the metadynamics technique.
Project C3 Complex Carbon Allotropes: Defected Graphenes, Composite Materials, and Topological Insulators by PI Shallcross will study how extended defects impact fundamental electronic properties of low dimensional carbon materials, as well as how such (unavoidable) defects may be controlled and utilized to tailor material properties, for example in plasmonics applications. A related line of investigation will study the interplay between the topological properties of Dirac-Weyl electrons and non-uniform strain in single and bilayer graphene systems. This will be studied in the context of novel composite materials formed of graphene and ordered arrays of metallic islands. Both of these projects are tightly integrated with experimental projects within which the electronic properties of extended defects and composite carbon materials form a focus of investigation. The theoretical methods to be developed and utilized will be based on low energy effective Hamiltonian descriptions of the complex graphene based materials.
Project C4 Theory and Simulation of Charge Transport and Light-Induced Processes in Carbon-Based Molecular Systems by PI Thoss will concentrate on the study of electron transport and photoinduced processes in molecular assemblies with synthetic carbon allotropes. Specific processes to be investigated, in close collaboration with the experimental projects, include charge transport in molecule-graphene nanojunctions, focusing on vibronic effects, spin-sensitive transport, light-driven transport phenomena, and photoinduced electron transfer in carbon-rich molecular assemblies. The theoretical methodology to be developed and used in this project will be based on a combination of first-principles electronic structure calculations and quantum dynamical as well as quantum transport approaches.
The four groups in Research Area C provide the broad expertise needed to handle the diverse questions posed in the experimental projects of Research Area A, B, and Z. Based on this expertise, theory and simulations will assist and guide the experimental work. Employing state-of-the-art methodology, the theory projects will provide information that often cannot be obtained easily from experiments, including electronic and geometric structure, energetics, stability of synthetic carbon allotropes, and analyses of reaction and transport mechanisms. In addition, the theory projects aim to initiate new research directions on the basis of theoretical predictions.
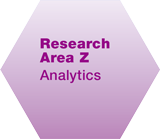
Project Z1 Gas-Phase Investigations of Synthetic Carbon Allotropes: Interaction, Reactivity, and Conversion by PI Drewello is concerned with gas-phase investigations of pure and derivatized synthetic carbon allotropes by advanced mass spectrometry. The project Z1 is mainly contributing to our focal topic: All-carbon architectures. Experiments in the dilute gas-phase allow the study of intrinsic properties of isolated species free from bulk or solvent effects. So-called soft ionization methods, such as Matrix-Assisted Laser Desorption/Ionization (MALDI) and Electrospray Ionization (ESI), that were tailored for the gentle ion formation of SCAs will be applied to the formation of only weakly bound aggregates and to the selective attachment of metal ions to SCAs. Direct Laser Desorption/Ionization (LDI) is employed as a harsh activation method to induce energy-demanding processes, such as coalescence of SCAs and/or cross-linking towards larger carbon architectures. A key experiment for the investigation of ions is tandem mass spectrometry (MS/MS, MS2) in which fragmentations of ions are promoted through collision (Collision-Induced Dissociation, CID). These experiments will be conducted on dedicated mass spectrometers and allow the elucidation of structures and relative bond strengths. Ion trap technology will be used to conduct MSn experiments, providing a fragmentation pedigree for n-1 generations of successive fragment ions of a chosen precursor ion. Hybrid quadrupole time-of-flight mass spectrometry allows to conduct energy-resolved CID, which enables the establishment of relative affinities and bond strengths.
The project Z1 proposes three work packages. WP-1 ”SCA Interaction“ investigates the non-covalent bonding between and to SCAs (p-p, ion/neutral bonding). The systems under investigation will include ligated azafullerenes (A1 Hirsch), porphyrins with carbon-rich peripheries such as modified HBCs (A2 Jux), heteroatom-doped PAHs (A5 Kivala) and C60@CPP in C60-endcapped rotaxanes (A7 von Delius) with CPP = cycloparaphenylene. WP-2 ”SCA reactivity“ proposes to investigate metal-induced reactions with polyynes and cumulenes as well as their respective rotaxanes. The reaction behavior will be studied for selected transition metal ions by variation of end group, nature, and length of the carbon chain and the nature and size of the macrocycle (A4 Tykwinski). Experiments will be accompanied by DFT theory (C2 Görling). WP-3 “SCA conversion“ investigates the formation of larger carbon architectures. This includes the mechanisms of cyclisation reactions by HF elimination based on porphyrin cores and related precursors (A2 Jux, A6 Amsharov). A second major part is concerned with the laser activation of carbyne prototypes (polyynes and cumulenes) investigating the process of conversion and their behavior towards aggregation and cross-linking, as well as the use of graphynes as target material for larger carbon architectures (A4 Tykwinski). Furthermore, Z1 is deeply embedded in Research Area C (C1 Clark/Meyer, C2 Görling) and linked to B10 Guldi.
Project Z2 Aberration-Corrected High Resolution and in situ Transmission Electron Microscopy of Carbon Allotropes and Related Device Structures by PIs Spiecker/Butz will employ the analytical and high-resolution capabilities of the new double aberration-corrected FEI Titan Themis3 300 microscope at the Center for Nanoanalysis and Electron Microscopy (CENEM) for detailed investigations of individual functionalized and non-functionalized synthetic carbon allotropes (collaboration with projects A1 Hirsch, A3 Peukert, A7 von Delius, B7 Steinrück/Papp, B10 Guldi), related composites (B3 Halik) as well as thin films and device structures ( A3 Peukert, B1 Brabec, B3 Halik, B8 Weber, B10 Guldi). The Titan Themis3, which replaced the former single (image-side) corrected Titan3 80-300 in 2014, is extensively equipped with latest technologies like high-brightness gun, monochromator, probe + image aberration corrector, ChemiSTEMTM (large-area X-ray detector, covered solid angle 0.7 srad), and high-resolution/high-speed Gatan Quantum energy filter. Apart from improved HRTEM imaging at low voltages (< 1 Å @ 60/80 kV) the new capabilities will be exploited for imaging and chemical analyses (EDXS, EELS) in STEM mode on the Å-scale, a major task in the focal topic Chemistry on Surfaces. Another important instrument, which has been installed at CENEM in 2013 and has already been used in numerous collaborations with SFB 953 groups is the FIB-SEM FEI Helios Nanolab 660 which is equipped with a monochromator for improved resolution at low electron energies. Continuing the work of the first funding period, this instrument will be extensively employed for analyzing cross-sections of thin films and devices and for preparing thin lamellae for advanced TEM characterization of interfaces and film structures (collaborations with A3 Peukert, B1 Brabec, B3 Halik, B8 Weber, B10 Guldi). In particular, combined FIB-SEM and TEM studies aim at contributing to an understanding of All-Carbon Architectures, one of the focal topics in the second funding period. As a major research focus project Z2 will contribute to the focal topic Structures and Properties of Extended Defects by further exploring the structure of dislocations and planar faults in bi-layer (and few-layer) graphene and their role for the mechanical, electrical, and chemical (functionalization) properties of such quasi-2D materials. In order to most efficiently address these aspects Z2 will closely collaborate with both experimental (A1 Hirsch, A3 Peukert, B4 Maier, B8 Weber) and modeling ( C1 Clark/Meyer, C3 Shallcross) groups which turned out to be very efficient in the first funding period. Lattice strain introduced by dislocations and membrane buckling resulting from strain relaxation will be studied experimentally by TEM, diffraction tomography as well as Raman microspectoscopy and will be complemented by atomistic simulations and DFT calculations in order to learn more about, e.g., the impact on surface functionalization and local band structure. Moreover, in situ nanomechanical testing inside the TEM and SEM will be carried out to understand the formation and dynamics of dislocations and to get an insight into the interface energies and defect processes that govern relative slip (and exfoliation) of graphene layers in by-layer (and few-layer) graphene.